CHAPTER:2 Avian behavior, form and function: Foraging and Flight
Identify characteristics of birds
LEARNING OBJECTIVES
- Identify characteristics of birds
- Describe the derived characteristics in birds that facilitate flight
- Describe the evolutionary history of birds
Characteristics of Birds
Birds are endothermic, and because they fly, they require large amounts of energy, necessitating a high metabolic rate. Like mammals, which are also endothermic, birds have an insulating covering that keeps heat in the body: feathers. Specialized feathers called down feathers are especially insulating, trapping air in spaces between each feather to decrease the rate of heat loss. Certain parts of a bird’s body are covered in down feathers, and the base of other feathers have a downy portion, whereas newly hatched birds are covered in down.
Figure 1. Primary feathers are located at thewing tip and provide thrust; secondary feathersare located close to the body and provide lift.
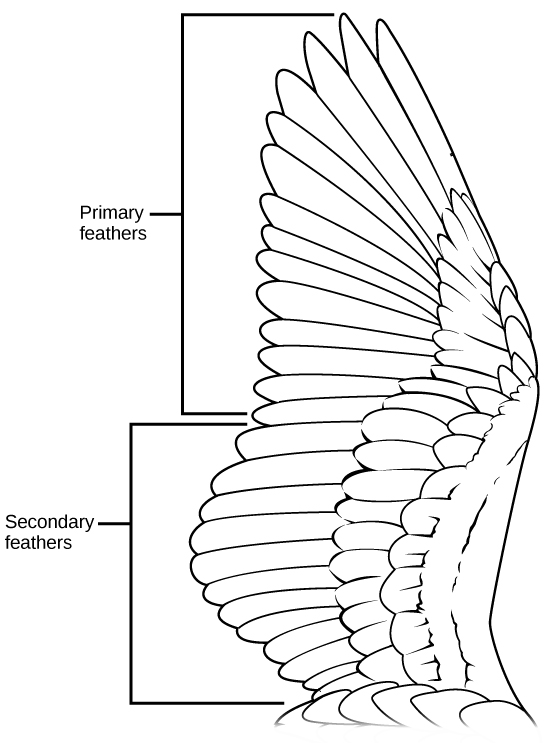
Feathers not only act as insulation but also allow for flight, enabling the lift and thrust necessary to become airborne. The feathers on a wing are flexible, so the collective feathers move and separate as air moves through them, reducing the drag on the wing. Flight feathers are asymmetrical, which affects airflow over them and provides some of the lifting and thrusting force required for flight (Figure 1). Two types of flight feathers are found on the wings, primary feathers and secondary feathers. Primary feathers are located at the tip of the wing and provide thrust. Secondary feathers are located closer to the body, attach to the forearm portion of the wing and provide lift. Contour feathers are the feathers found on the body, and they help reduce drag produced by wind resistance during flight. They create a smooth, aerodynamic surface so that air moves smoothly over the bird’s body, allowing for efficient flight.
Flapping of the entire wing occurs primarily through the actions of the chest muscles, the pectoralis and the supracoracoideus. These muscles are highly developed in birds and account for a higher percentage of body mass than in most mammals. These attach to a blade-shaped keel, like that of a boat, located on the sternum. The sternum of birds is larger than that of other vertebrates, which accommodates the large muscles required to generate enough upward force to generate lift with the flapping of the wings. Another skeletal modification found in most birds is the fusion of the two clavicles (collarbones), forming the furcula or wishbone. The furcula is flexible enough to bend and provide support to the shoulder girdle during flapping.
An important requirement of flight is a low body weight. As body weight increases, the muscle output required for flying increases. The largest living bird is the ostrich, and while it is much smaller than the largest mammals, it is flightless. For birds that do fly, reduction in body weight makes flight easier. Several modifications are found in birds to reduce body weight, including pneumatization of bones. Pneumatic bones are bones that are hollow, rather than filled with tissue (Figure 2). They contain air spaces that are sometimes connected to air sacs, and they have struts of bone to provide structural reinforcement. Pneumatic bones are not found in all birds, and they are more extensive in large birds than in small birds. Not all bones of the skeleton are pneumatic, although the skulls of almost all birds are.
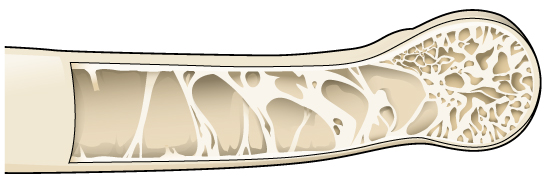
Figure 2. Many birds have hollow, pneumatic bones, which make flight easier.
Other modifications that reduce weight include the lack of a urinary bladder. Birds possess a cloaca, a structure that allows water to be reabsorbed from waste back into the bloodstream. Uric acid is not expelled as a liquid but is concentrated into urate salts, which are expelled along with fecal matter. In this way, water is not held in the urinary bladder, which would increase body weight. Most bird species only possess one ovary rather than two, further reducing body mass.
The air sacs that extend into bones to form pneumatic bones also join with the lungs and function in respiration. Unlike mammalian lungs in which air flows in two directions, as it is breathed in and out, airflow through bird lungs travels in one direction (Figure 3). Air sacs allow for this unidirectional airflow, which also creates a cross-current exchange system with the blood. In a cross-current or counter-current system, the air flows in one direction and the blood flows in the opposite direction, creating a very efficient means of gas exchange.
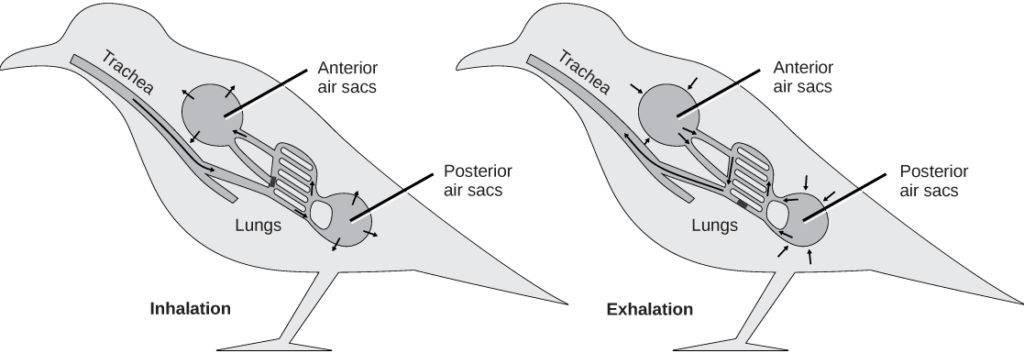
Figure 3. Avian respiration is an efficient system of gas exchange with air flowing unidirectionally. During inhalation, air passes from the trachea into posterior air sacs, then through the lungs to anterior air sacs. The air sacs are connected to the hollow interior of bones. During exhalation, air from air sacs passes into the lungs and out the trachea. (credit: modification of work by L. Shyamal)
IN SUMMARY: CHARACTERISTICS OF BIRDS
Birds are endothermic, meaning they produce their own body heat and regulate their internal temperature independently of the external temperature. Feathers not only act as insulation but also allow for flight, providing lift with secondary feathers and thrust with primary feathers. Pneumatic bones are bones that are hollow rather than filled with tissue, containing air spaces that are sometimes connected to air sacs. Airflow through bird lungs travels in one direction, creating a cross-current exchange with the blood.
Origin of FlightExactly how birds acquired the ability to fly has baffled scientists for years. Archaeopteryx provided a starting point for speculation. Built like a dinosaur, but with wings, scientists guessed at how a hypothetical ancestor might have taken flight. Some scientists support the arboreal hypothesis (e.g., Feduccia 1996) and suggest that the ancestors of Archaeopteryx lived in trees and glided into flapping flight (Figure to the right).
But others argue that the claws of Archaeopteryx weren't suited to climbing. So, others support the cursorial hypothesis (e.g., Burgers and Chiappe 1999) and suggest that these ancestors used their long, powerful legs to run fast with their arms outstretched, and were at some point lifted up by air currents and carried into flapping flight (Figure to the bottom right). Studying living animals can throw light on their evolutionary past. Ken Dial (2003) of the Flight Lab at the University of Montana noticed the ability of gamebird chicks to escape danger by scrambling up vertical surfaces. The chicks first run very fast, flapping their immature, partially feathered wings, frantically creating enough momentum to run up a vertical surface to safety. Could this survival instinct be the origin of flight? And finally, James Carey, a UC Davis demographer and ecologist, has proposed that the evolution of bird flight is linked to parental care (Carey and Adams 2001). Whatever the origins, dinosaurs, and birds, eventually took to the air. Images & text used with permission. © The Natural History Museum, London - www.nhm.ac.uk |
Dinosaurs' flapping led to flight? The feathered forelimbs of small, two-legged dinosaurs may have helped them run up hills or other inclines to escape predators. This half running, half flapping may have evolved into an ability to fly. Dial (2003) reported findings suggesting that the ability to fly evolved gradually. Feathers may have first protected animals from cold & wet weather, then been used out of necessity when something with big teeth was chasing them. Even before their wings develop enough to fly, some living birds use them to improve traction and gain speed. Dial studied birds, like partridges, capable of only limited flight. Energetically, "It's a lot cheaper to run than fly," Dial said. So these baby birds, with big feet & powerful legs, use them in combination with their wings, first to stay balanced & grounded, then to take on steeper and steeper inclines. Using this "wing assisted incline running," Chukar Partridges can negotiate 50 degree inclines right after hatching, 60 degree slopes at 4 days old, and at 20 days, can perform a vertical ascent. "The wings help them stick to the ground," said Dial. The wings only come into play on steep angles because at about a 50 - 60 degree incline the birds start slipping. Then they begin a head to tail movement, like a reptile, that pushes them to the ground to enhance traction. "They use their wings like spoilers on a race car, to give their feet better traction," he said. Use of this wing-assisted running doesn't stop when the birds are old enough to fly. Adult birds often choose the running and flapping option instead of flying because it is more energy efficient. - Written by Marsha Walton, CNN |
Flight requires lift, which results when an air stream passing over wing must travel further (and faster) than the air stream passing under a wing. The slower moving air under the wing 'pushes' against the bottom of the wing with greater force than the faster moving air above the wing & this generates lift. The lift generated is influenced by a bird's velocity (See http://www.grc.nasa.gov/WWW/K-12/airplane/vel.html).
Why does the slower moving air generate more pressure against the wing than the faster moving air? In calm air, the molecules are moving randomly in all directions. However, when air begins to move, most (but not all) molecules are moving in the same direction. The faster the air moves, the greater the number of air molecules moving in the same direction. So, air moving a bit slower will have more molecules moving in other directions. In the case of a wing, because air under the wing is moving a bit slower than air over the wing, more air molecules will be striking the bottom of the wing than will be striking the top of the wing. This is called the Bernoulli effect & this creates lift!
When the curvature over the top becomes greater by increasing the angle of attack (below), the air moves even faster over the top of the wing and more lift is generated. Eventually, however, if the angle of attack becomes too great, the flow separates off the wing and less lift is generated. The result is stalling. For birds, the optimum angle of attack is typically about 3 - 5 degrees. Birds also tend to stall at low speeds because slower moving air may not move smoothly over the wing.
Source: http://courses.egr.duke.edu/PROJECTS/Modules/mammals/lesson/wingskeleton.html | Source: http://www.windsofkansas.com/fltphoto.html |
When these feathers are elevated (above right & below right), they keep air moving smoothly over the wing & help a bird maintain lift.
Source: http://www.lam.mus.ca.us/birds/guide/pg018.html
The fossilized remains of a tiny bird provides evidence that birds flew as nimbly 115 million years ago as their descendants do today. The fossilized bird, Eoalulavis hoyasi, was found in a limestone quarry in Spain (Sanz et al. 1996). About the size of a goldfinch, the bird had an alula, or bastard wing, that would have helped it stay aloft at slow speeds. Eoalulavis is the most primitive bird known with an alula. Eoalulavis hoyasi. Top, fluorescence induced ultraviolet photo of the specimen before preparation. Bottom, reconstruction. A - alula, PR - primary remiges, and SR - secondary remiges (Sanz and Ortega 2002). Archaeopteryx probably flapped and glided, but did not have an alula. Eoalulavis provides evidence that by 30 million years after Archaeopteryx, at least one group of early birds had developed the alula. Eoalulavis hoyasi, which means "dawn bird with a bastard wing from Las Hoyas," was discovered at a site where a freshwater lake existed millions of years ago. The bird may have hunted by wading in shallow water the way plovers and other shorebirds do today. |
Of course, a wing moving through the air is opposed by friction & this is called drag. The two main types of drag acting on birds are pressure (or induced) drag & friction (or profile) drag. Induced drag occurs when the air flow separates from the surface of a wing, while friction drag is due to the friction between the air and bird moving through the air. Friction drag is minimized by a wing's thin leading edge (wings 'slice' through the air). Induced drag occurs at low speeds and at higher speeds as, at wing tips, air moves from the area of high pressure (under the wing) to the area of low pressure (top of the wing). As wings move through the air, this curling action causes spirals (vortices) of air (see photo of continuous vortices to the right) which can disrupt the smooth flow of air over a wing (and reduce lift). |
(A) Depictions of the vortex-ring and continuous-vortex gaits. (B) Cross-sectional view of the wing profile. Lift produced during flapping provides weight support (upward force) and thrust (horizontal force). In the vortex-ring gait, lift is produced only during the downstroke, providing positive upward force and forward thrust. In the continuous-vortex gait, lift is produced during both the upstroke and the downstroke. The downstroke produces a positive upward force and forward thrust; the upstroke produces a positive upward force and rearward thrust. Partial flexion of the wing during the upstroke reduces the magnitude of the rearward thrust to less than that of the forward thrust produced during the downstroke, providing net positive thrust per wingbeat (From Hedrick et al. 2002). |
Birds are known to employ two different gaits in flapping flight, a vortex-ring gait in slow flight and a continuous-vortex gait in fast flight. In the vortex ring gait, the upstroke is aerodynamically passive (there is no bound circulation during this phase, and hence no trailing vortex), and the wings flex and move close to the body to minimize drag. In the continuous vortex gait (where each wingtip sheds a separate vortex trail during both the upstroke and downstroke), the wings are aerodynamically active throughout (i.e., lift is generated both during the downstroke and the upstroke), while the wings remain near-planar throughout and deform only by flexure at the wrist. Hedrick et al. (2002) studied the use of these gaits over a wide range of speeds in Cockatiels and Ringed Turtle-doves trained to fly in a wind tunnel. Despite differences in wing shape and wing loading, both species shifted from a vortex-ring to a continuous-vortex gait at a speed of 7 meters/sec. They found that the shift from a vortex-ring to a continuous-vortex gait depended on sufficient forward velocity to provide airflow over the wing during the upstroke similar to that during the downstroke. This shift in flight gait appeared to reflect the need to minimize drag and produce forward thrust in order to fly at high speed. |
A convenient way to describe the shape of a wing is by its aspect ratio - the ratio of length to width. Among bird wings, aspect ratios vary from about 1.5 to as high as about 18. Elliptical (or 'rapid takeoff') wings (above) have relatively low aspect ratios, while high speed wings & soaring wings have high aspect ratios.
- The long (or soaring) wings of birds with very high aspect ratios, like albatrosses, generate lots of lift, while the narrow, pointed shape helps reduce drag while gliding (because the small area of the pointed tip minimizes pressure differences and, therefore, turbulence at the wing tip).
Laysan Albatross wing (Source: http://www.ups.edu/biology/museum/wingphotos.html)
Whistle through the wing Wilson's Storm Petrel Photo by Brian Patteson http://www.patteson.com/image2.htm Birds molt for a variety of reasons. Molting regulates body temperature, keeps feathers neat and waterproof and allows seasonal changes in appearance for mating or migration. However, generating new feathers uses extra energy; staying warm with less plumage uses extra energy, and flying with smaller, work-in-progress wings requires extra energy. So, not all birds molt in the same way. Ducks, swans and geese, for example, shed all their flight feathers at once and are flightless until replacements have grown. Most other birds, however, lose and renew their feathers according to a continuous, pre-programmed sequence. This sequential molting gives rise to a range of temporary feather gaps that seem to reduce take-off speed, take-off angle and level flight speed and to impede predator evasion by raising a bird's minimum turning radius. Anders Hedenstrom and Shigeru Sunada of Cambridge University estimated how the aerodynamics of flight are affected by molting (Hedenstrom & Sunada 1999). They estimated drag and lift by analyzing the fluid dynamics of symmetrical gaps in flat, rectangular model wings of various width-to-length (aspect) ratios, at a fixed angle with respect to air flow – a system that reasonably approximates a bird in gliding, but not flapping, flight. Although the effects were small, Hedenstrom and Sunada concluded that both feather gap size and position affect flight performance. Large gaps, and gaps in the middle of the wing impede aerodynamic efficiency more than small, wing-tip gaps. They also found that the detrimental effect of molt gaps increases with increasing aspect ratio. In other words a bird with short, broad wings, like a vulture, won't miss a few feathers as much as one with long, narrow wings, like an albatross. "This is of great ecological significance," they muse, "as it could help explain why large birds show relatively slow rates of molting that are associated with rather small gaps." -- Sara Abdulla, Nature Science Update |
- High speed wings, like those of falcons, swallows, & swifts, have relatively high aspect ratios. These narrow, tapering wings can be flapped rapidly to generate lots of speed with minimal drag (because, again, the small area of the pointed tip minimizes pressure differences and turbulence at the wing tip).
Chimney Swift wing (Source: http://www.ups.edu/biology/museum/wingphotos.html)
How pigeons give falcons the slip -- A Peregrine Falcon dive-bombing at several hundred miles an hour to knock a pigeon out of the sky would seem to be a study in single-mindedness. At those speeds, attention must be paid. But even a falcon in hot pursuit can become distracted. And what distracts it, is a patch of white feathers on the rump of an otherwise blue-gray pigeon. "The brain can be primed by a conspicuous thing," said Alberto Palleroni. The falcon, he said, sees the conspicuous thing -- the white patch -- and doesn't notice the pigeon starting to turn away and escape. "In effect, it's a kind of a card trick or a ruse" on the part of the pigeon, Palleroni said.
Palleroni et al. (2005) observed more than 1,800 falcon attacks on wild pigeons over seven years. They recorded the plumage types among the pigeons and noticed that while birds with white rump patches made up 20% of the pigeon population, very few were captured by the falcons. When a Peregrine Falcon attacks a pigeon, it plunges at speeds greater than 200 miles an hour, levels off and comes upon the pigeon from behind, punching it with what amounts to a closed fist. At those speeds even a grazing blow kills the pigeon; the falcon then circles back and picks it up. The only way the slower-flying pigeon can escape is by dipping a wing, rolling and veering off. If the falcon is distracted by the white patch, it won't notice the dipping of the wing (which, being blue-gray, blends with the landscape) until it's too late. Plumage color in pigeons is an independently heritable trait, Palleroni said, meaning it is not tied to selection involving sexual or other traits. So it is highly likely that the white rump feathers are an anti-predator adaptation to high-speed attacks. Not bad for a bird that many people disdain. "The feral pigeon is an amazing balance of adaptations and success," Palleroni said. - Henry Fountain, New York Times |
- High-lift wings have lower aspect ratios & there are spaces between the feathers at the end of the wing. These 'slots' help reduce drag at slow speeds because the separated tip feathers (shown in the White Stork pictured below) act as 'winglets' and spread vorticity both horizontally and vertically.
Check out Discovery's bird-tech site with video shots from tiny cameras attached to Tilly the Golden Eagle.
- Wings with low aspect ratios (elliptical wings), like those of many songbirds, woodpeckers, pheasants, & quail, permit sharp turns while flying among trees & shrubs.
Spruce Grouse wing (Source: http://www.ups.edu/biology/museum/wingphotos.html)
The Flight Strategy of Magnificent Frigatebirds -- Frigatebirds cannot land on the sea because their feathers are not waterproof. If they did land, they would find it even harder to take off again because their legs are too short. Despite this, frigatebirds are perfectly suited for an aerial life over the sea because they have the lowest wing-loading (large wing area & low body mass) of any bird. Weimerskirch et al. (2003) investigated the movements of Magnificent Frigatebirds (Fregata magnificens) while foraging at sea off the coast of French Guiana. Because they are very light in comparison to their wing surface, frigatebirds can glide at altitudes of up to 2,500 meters. Then they glide downward, taking advantage of the next current. This flight strategy, which limits the bird's physical efforts, is the same as that used by migratory birds during long flights over land. Migratory birds, however, avoid flying over the sea due to a lack of thermals, while frigatebirds fly over the sea. As it turns out, ascending air currents are found over the sea only in tropical regions where the waters are warm enough to create such currents on a continuous basis. Frigatebirds can therefore fly night and day using this technique. To investigate the movements of Magnificent Frigatebirds, Weimerskirch et al. (2003) fitted the birds with satellite transmitters and altimeters, which allowed them to observe that the birds only occasionally come close to the sea surface to catch prey. They catch flying fish or squid driven above the surface by underwater predators like schools of tuna or dolphins. To identify such feeding opportunities, which are very rare, requires long hours of flight at high altitudes. |
The high wing loading of birds like grebes, loons (check Looney Lift-Off), and swans (see Tundra Swan below) means that it's more difficult for them to generate sufficient lift to take-off. That's why these birds often run along the surface of a lake for some distance before taking flight. They must generate enough speed to generate enough lift to get their relatively heavy bodies into the air!
Want to see a Laysan Albatross taking flight?? Check this video!
Landing - Birds must usually be much more precise when landing than an airplane pilot; often landing on a branch rather than a runway. |
When gliding, a Common Swift shows a torpedo-shaped body. Its arm-wing (close to its body) has a rounded leading edge.The bird's long, slender hand-wing has a much sharper profile. The inset shows the feathers at the hand-wing's leading edge. Birds have two-part wings. The proximal "arm wing" is rounded on front, humped on top, and sharp on the back -- just like most airplane wings. Further away, the "hand wing" is flatter on top and extremely sharp on the front. The hand wing resembles the wing of a fighter plane, and it is also often swept back -- angled -- toward the rear. Wings on some high-performance jets can change angle to alter the leading-edge vortex. Wings that are nearly straight out create more lift. Swept-back wings create more drag (air friction). Acrobatic birds may also take advantage of the LEV; changing wing angle gives them the ratio of lift and drag they need for flying and snatching insects in mid-air. The LEV not only creates lift, especially at slow speeds, but also confers another benefit that helps the swift perform insectivorous aerobatics. While conventional lift is chiefly an upward force, the LEV can also produce drag, which allows sudden steering. "The LEV can be used for controlling flight," says Videler. "It's very suited for that because there is no time delay, the forces are produced instantaneously. That's very useful if you want to maneuver very quickly." -- Courtesy of the University of Wisconsin Board of Regents |
0 Comments